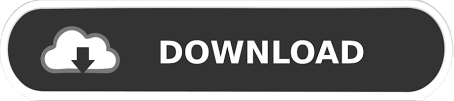

Coordination effect assisted synthesis of ultrathin Pt layers on second metal nanocrystals as efficient oxygen reduction electrocatalysts. Inflating hollow nanocrystals through a repeated Kirkendall cavitation process. Electrocatalysis on bimetallic surfaces: modifying catalytic reactivity for oxygen reduction by voltammetric surface dealloying.
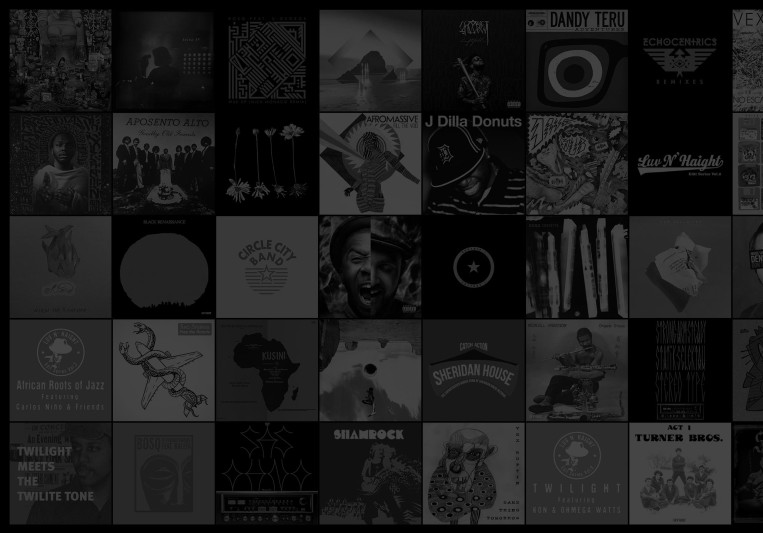
Atomic layer-by-layer deposition of Pt on Pd nanocubes for catalysts with enhanced activity and durability toward oxygen reduction. Direct and continuous strain control of catalysts with tunable battery electrode materials. Palladium-platinum core–shell icosahedra with substantially enhanced activity and durability towards oxygen reduction. Biaxially strained PtPb/Pt core/shell nanoplate boosts oxygen reduction catalysis. Surface lattice-engineered bimetallic nanoparticles and their catalytic properties. Lattice-strain control of the activity in dealloyed core–shell fuel cell catalysts. Stability and ORR performance of a well-defined bimetallic Ag 70Pt 30/Pt(111) monolayer surface alloy electrode-probing the de-alloying at an atomic scale. Theoretical surface science and catalysis-calculations and concepts. Effect of strain on the reactivity of metal surfaces. Platinum-based nanocages with subnanometer-thick walls and well-defined, controllable facets. Single-atom tailoring of platinum nanocatalysts for high-performance multifunctional electrocatalysis. PdMo bimetallene for oxygen reduction catalysis. We anticipate that our approach can be used to screen out lattice strain that will optimize the performance of Pt catalysts-and potentially other metal catalysts-for a wide range of reactions. We use this strain control to tune the electrocatalytic activity of the Pt shells over a wide range, finding that the strain–activity correlation for the methanol oxidation reaction and hydrogen evolution reaction follows an M-shaped curve and a volcano-shaped curve, respectively. Here we show that when ultrathin Pt shells are deposited on palladium-based nanocubes, expansion and shrinkage of the nanocubes through phosphorization and dephosphorization induces strain in the Pt(100) lattice that can be adjusted from −5.1 per cent to 5.9 per cent.

However, it is challenging to map in detail the strain–activity correlations in Pt-catalysed conversions, which can involve a number of distinct processes, and to identify the optimal strain modification for specific reactions. This dependence can be exploited for catalyst design 4, 6, 7, 8, and the use of core–shell structures and elastic substrates has resulted in strain-engineered Pt catalysts with drastically improved electrocatalytic performances 7, 9, 10, 11, 12, 13. The activity of Pt is controlled by its electronic structure (typically, the d-band centre), which depends sensitively on lattice strain 4, 5. Platinum (Pt) has found wide use as an electrocatalyst for sustainable energy conversion systems 1, 2, 3.
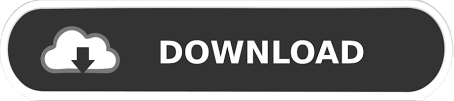